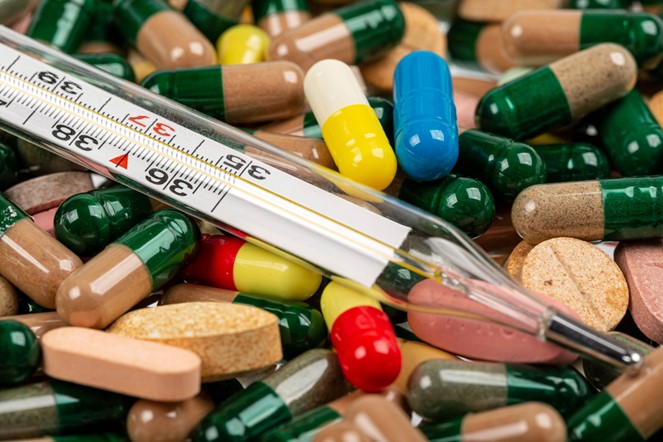
Steps to Discover Which Biotech Stocks May Get Hot
The biotech sector is in and of itself exciting. A company developing an idea that can improve human lives, decrease suffering, or even prevent death, by nature, could be a more rewarding endeavor than investing in a company that, by comparison, does little to make a big difference. If, at the same time, the opportunity to return the investor a multiple over returns available elsewhere in the market, then the motivation to allocate a portion of investment capital increases dramatically. But how does an investor gauge a company in the biotech sector and evaluate its chance of survival and likelihood of success?
As with much of investing, sure things don’t often provide a good return. And adding risk doesn’t necessarily equate to added return. The higher perceived risk of a sector such as biotech needs to be offset by research. Filtering stocks through a selection process is key, so the probability of picking those that survive and thrive is higher than average.
I spoke with Robert LeBoyer, the Senior Life Sciences Analyst at Noble Capital Markets, and asked him to list factors to improve the likelihood of choosing a successful biotech company. His knowledge and enthusiasm for the sector caused me to want to share what I learned.
Differentiation
Companies developing a drug that is different than all that came before for what it proposes to treat or prevent stand a good chance of getting funding to make it through the different phases of study. With a development time of 3-5 years, it is best if there is a clear unmet need for the therapy or no current therapies at all.
Investors should determine if there are treatments presently and ask whether the drug or treatment mechanism is a significant improvement over any current product. Also is the field crowded or will it soon be crowded with alternatives to what a company may bring to market? LeBoyer recommended asking where there is an improvement. He gave the example of many cholesterol-lowering drugs, which he said all target the same enzyme. A company with a drug that similarly targets that enzyme may not be worth exploring. Learning of a company that has a different mechanism of action, one which shows promise of greater efficacy, or, reduced cost, or fewer side effects may be worth exploring further.
As an example of a company that had met these criteria some years back, Gilead received approval for a once-daily tablet to treat hepatitis C. Prior to this, the only treatment options involved a year-long regimen of weekly interferon injections and ribavirin tablets. The side effects were depression, nausea, flu-like symptoms, and a reduction in some blood cells. The cost of the injections and treatments could cost a health insurer $1 million over the life of the patient. The Gilead treatment, which has a price tag of $93,000, is mathematically more cost-effective. The therapy which Gilead got approved in the U.S. in 2013 was a better treatment than what existed, and even better tolerated by patients. The stock went from $20 to $120 in about a years time after approval.
Development
Clinical development was another attribute brought up by Noble’s analyst. With even the best proof of concept or early-stage trial success, assessing the chance that clinical stage trials may fail for pipeline candidates is difficult. This is why a company with a diverse pipeline with a number of products being developed or in later stages of clinical trials, increases the probability of successful biotech investment. Many companies easily pass stage one trials and even stage two, but don’t get past the final hurdle. LeBoyer shared with me a story of a company he now covers that had a vaccine for Covid-19 early on. The human clinical trials, however, were not done in the U.S., but were instead the result of trials on persons mostly of similar lineage. The FDA required a sampling comparable to the diversification of heritage or gene pool in the U.S.
Obtaining a basic understanding of the FDA side of development is important for anyone making decisions on biotech stocks.
The drug approval process in the U.S. involves multi-layered (Step One through Step Four) with each representing an important milestone on the path to full approval so the product can be brought to market or meet rejection along the path.
Step One is the development phase, Step Two is research, Step Three is Clinical Trials, and Step Four is FDA Approval.
Knowing where companies stands in the FDA step process can help an investor assess the likelihood of approval. Many products, can get to the last step and not be approved, but those just starting out on Step One are a greater risk both in the time it will take and the chance for something to not be to the FDA’s liking.
Finances
Biotech companies, by and large burn through cash in their research, development and trial periods. Understanding how long the cash on hand and other available sources can last before they need to raise more cash, then comparing this with how close to an expected finish line they are, could help an investor steer away from a company that may have a product in the pipeline that meets other key elements investors should look at, but unfortunately, funds may stand in the way of success.
Robert LeBoyer explained that the current high-interest rate environment, coupled with depressed stock prices, makes this particularly important now. For those companies that can borrow, the cost of money is now far more expensive than it had been in the last decade and a half. And issuing more shares, essentially selling more of the company, dilutes the value of shares currently held. It could become a tricky situation that stockholders or those deciding to become a stockholder should monitor
Take Away
Are there companies with a pipeline that includes drugs that meet a large unmet need (as one example, Alzheimer’s), or can attack a disease like cancer in a unique way that would be embraced by the medical community and patients? “Unicorn” companies do exist, but finding them, assessing them, and doing it before a louder investment buzz occurs takes some digging. A solid place to start digging is under the biotech company section on Channelchek. Available by clicking here, here investors are exposed to many opportunities and the underlying data, the latest news, and of course, thorough company descriptions.
Biotech companies covered by analyst Robert LeBoyer, along with his current research are available here. Channelchek will be highlighting interesting biotechs in future articles and discussing their work and status against the criteria presented above. Join Channelchek to receive emails and gain free access to these articles, video presentations, updated research reports, and news of company roadshows. Visit Rob LeBoyers coverage list here.
Managing Editor, Channelchek